Unveiling The Secrets: What Is The Temperature Of The Core Of The Sun?
Have you ever wondered what powers the immense energy of the Sun, the very source of life on Earth? At its heart lies a fiery core, a region so hot that it defies human imagination. What is the temperature of the core of the Sun, you might ask? Scientists estimate that it reaches a staggering 15 million degrees Celsius (27 million degrees Fahrenheit). This unimaginable heat is the engine behind nuclear fusion, the process that fuels our star and sustains life across the solar system. Understanding the core's temperature is not just a scientific curiosity but a key to unlocking the mysteries of the universe.
The Sun's core is an extraordinary environment where hydrogen atoms fuse to form helium, releasing vast amounts of energy in the process. This energy eventually radiates outward, taking thousands of years to reach the surface and then traveling across space to warm our planet. The temperature of the Sun's core is a critical factor in this process, as it determines the rate of nuclear fusion. Without this immense heat, the Sun would not shine, and life as we know it would cease to exist. But how do scientists even measure something so far away and so intensely hot?
Studying the Sun's core has been a monumental challenge for astronomers and physicists. Since we cannot directly observe the core, scientists rely on indirect methods, such as analyzing solar vibrations and modeling the Sun's internal structure. These efforts have allowed us to estimate the core's temperature with remarkable accuracy, shedding light on the fundamental processes that govern stars. As we dive deeper into this topic, we will explore not only the temperature of the Sun's core but also its implications for science, technology, and our understanding of the cosmos.
Read also:Discovering The Impact Of Fox Lisa Boothe A Comprehensive Guide
Table of Contents
- What Makes the Sun's Core So Hot?
- How Do Scientists Measure the Core Temperature?
- Why Is the Core Temperature Important for Life on Earth?
- The Nuclear Fusion Process: What Happens Inside the Sun?
- How Does the Core Affect the Sun's Other Layers?
- What Happens If the Sun's Core Cools Down?
- How Does the Sun's Core Compare to Other Stars?
- What Lies Ahead: Future Research and Discoveries?
What Makes the Sun's Core So Hot?
The Sun's core is an extraordinary furnace, where temperatures soar to unimaginable heights. But what exactly causes this immense heat? At the heart of the matter is gravity. The Sun's immense mass—approximately 333,000 times that of Earth—creates a gravitational force so powerful that it compresses the core's material into an incredibly dense state. This compression generates extreme pressure, which in turn raises the temperature to levels that are difficult to comprehend.
Under these conditions, hydrogen atoms are stripped of their electrons, creating a plasma—a state of matter where particles move at incredible speeds. The pressure and density in the core are so high that hydrogen nuclei (protons) are forced to collide with one another. These collisions are the first step in the nuclear fusion process, where hydrogen atoms fuse to form helium. This process releases an enormous amount of energy in the form of light and heat, sustaining the Sun's brilliance and maintaining its core temperature at approximately 15 million degrees Celsius.
But why does this process occur only in the core? The answer lies in the Sun's layered structure. The core is the only region with sufficient temperature and pressure to overcome the natural repulsion between positively charged protons. Outside the core, conditions are not extreme enough to sustain nuclear fusion, which is why energy production is confined to this central region. This delicate balance of gravity, pressure, and temperature is what makes the Sun's core so uniquely hot and vital to the solar system's existence.
How Do Scientists Measure the Core Temperature?
Given that the Sun's core is located 696,000 kilometers beneath its surface, directly measuring its temperature is impossible with current technology. So, how do scientists estimate what is the temperature of the core of the Sun? The answer lies in a combination of theoretical models, observational data, and advanced techniques like helioseismology.
Helioseismology is the study of the Sun's vibrations, akin to listening to its "heartbeat." These vibrations, caused by pressure waves traveling through the Sun, provide clues about the star's internal structure. By analyzing these oscillations, scientists can infer the density, pressure, and temperature gradients within the Sun. These measurements are then used to refine computer models that simulate the Sun's core conditions.
What Role Do Solar Neutrinos Play?
Another critical tool in understanding the Sun's core is the detection of solar neutrinos. These elusive particles are byproducts of nuclear fusion and can escape the Sun's interior without interacting with its outer layers. By capturing and analyzing neutrinos using specialized detectors on Earth, scientists gain direct insight into the fusion processes occurring in the core. The energy distribution of these neutrinos helps confirm the estimated core temperature of 15 million degrees Celsius.
Read also:Scarlett Johansson Height And Weight A Comprehensive Guide To Her Life And Career
How Accurate Are the Models?
While models and indirect methods have provided reliable estimates, they are not without limitations. Uncertainties arise from assumptions about the Sun's composition and the behavior of plasma under extreme conditions. However, advancements in technology, such as more sensitive neutrino detectors and improved computational models, continue to enhance the accuracy of these measurements. The combination of multiple approaches ensures that scientists can confidently estimate the core's temperature, even without direct access to it.
Why Is the Core Temperature Important for Life on Earth?
The Sun's core temperature is not just a fascinating scientific fact; it is a cornerstone of life on Earth. The immense heat and pressure within the core drive the nuclear fusion process, which produces the energy that sustains our planet. This energy, radiated as sunlight, is the primary source of heat and light for Earth. Without the core's extreme temperature, the Sun would not generate enough energy to support life as we know it.
The stability of the Sun's core temperature is equally crucial. If the core were significantly hotter or cooler, the rate of nuclear fusion would change, leading to fluctuations in the Sun's energy output. A hotter core would accelerate fusion, potentially causing the Sun to burn through its hydrogen fuel too quickly. Conversely, a cooler core would slow fusion, reducing the Sun's energy output and plunging Earth into a deep freeze. This delicate balance ensures that the Sun remains a stable and reliable source of energy over billions of years.
Moreover, the core's temperature influences the Sun's lifecycle and its eventual fate. Understanding this temperature helps scientists predict how the Sun will evolve, including its transition into a red giant and its eventual collapse into a white dwarf. These predictions are vital for understanding the long-term future of our solar system and the potential impact on Earth. In essence, the core's temperature is not just a scientific curiosity but a fundamental factor in the story of life, the universe, and everything in between.
The Nuclear Fusion Process: What Happens Inside the Sun?
At the heart of the Sun lies the nuclear fusion process, a phenomenon that transforms hydrogen into helium and releases colossal amounts of energy. This process is the reason the Sun shines and the source of the heat and light that sustain life on Earth. But what exactly happens during nuclear fusion, and how does the core's temperature facilitate this reaction?
Nuclear fusion begins when hydrogen nuclei, or protons, collide under the extreme conditions of the Sun's core. The core's temperature of 15 million degrees Celsius provides the energy needed to overcome the electrostatic repulsion between these positively charged particles. Once the protons are close enough, the strong nuclear force takes over, binding them together to form a helium nucleus. This transformation is not instantaneous; it occurs through a series of steps known as the proton-proton chain reaction.
What Are the Steps of the Proton-Proton Chain Reaction?
- Step 1: Two protons fuse to form a deuterium nucleus (one proton and one neutron), releasing a positron and a neutrino in the process.
- Step 2: The deuterium nucleus collides with another proton to form helium-3, releasing a gamma-ray photon.
- Step 3: Two helium-3 nuclei combine to form a helium-4 nucleus, ejecting two protons in the process.
Each step releases energy in the form of photons, neutrinos, and other particles. These photons eventually make their way to the Sun's surface, where they are emitted as sunlight. The entire process is incredibly efficient, converting about 0.7% of the mass involved into energy, as described by Einstein's famous equation, E=mc².
The Sun's core is uniquely suited for this process due to its extreme temperature and pressure. These conditions ensure that hydrogen nuclei collide frequently enough to sustain fusion, while the immense pressure prevents the core from expanding and cooling. Without this perfect balance, the Sun would not be able to produce the energy required to power our solar system.
How Does the Core Affect the Sun's Other Layers?
The Sun's core does not operate in isolation; it profoundly influences the behavior and characteristics of the surrounding layers. These layers—comprising the radiative zone, the convective zone, and the photosphere—each play a role in transporting the energy produced in the core to the surface. But how exactly does the core's temperature and activity shape these layers?
The energy generated in the core radiates outward into the radiative zone, a region where photons travel in a zigzag pattern, bouncing off particles and gradually making their way toward the surface. The core's immense temperature ensures that this energy is intense enough to sustain this slow, million-year journey. Without the core's high energy output, the radiative zone would lack the thermal pressure needed to counteract gravitational collapse, potentially destabilizing the Sun's structure.
Further out, the convective zone operates under the influence of the core's energy as well. Here, hot plasma rises toward the surface, cools, and sinks back down in a continuous cycle. This convection is driven by the temperature gradient established by the core, which creates the necessary heat differential. The convective zone's activity also generates the Sun's magnetic field, which influences phenomena like sunspots and solar flares.
What Happens When the Core's Influence Weakens?
If the core's temperature were to decrease, the energy transported to the outer layers would diminish, leading to a cascade of effects. The radiative zone would cool, reducing the thermal pressure that supports the Sun against gravity. This could cause the Sun to contract, further disrupting the delicate balance of forces. Similarly, the convective zone might become less active, weakening the magnetic field and altering the Sun's behavior. In short, the core's temperature is the linchpin that holds the Sun's structure and dynamics together.
What Happens If the Sun's Core Cools Down?
While the Sun's core is currently a fiery powerhouse, it is not immune to change. Over billions of years, the core's temperature will gradually decrease as hydrogen fuel is depleted. But what happens if the core cools down significantly? The consequences would be profound, not only for the Sun itself but also for the entire solar system.
A cooling core would slow the rate of nuclear fusion, reducing the Sun's energy output. This decline in energy would cause the outer layers of the Sun to contract under the force of gravity. As the core continues to cool, the Sun would eventually exhaust its hydrogen fuel and transition into the next phase of its lifecycle: the red giant stage. During this phase, the core's contraction would heat the surrounding layers, igniting helium fusion and causing the Sun to expand dramatically.
If the core were to cool completely, fusion would cease entirely. Without the outward pressure generated by fusion, the Sun would collapse under its own gravity, ultimately forming a white dwarf. This dense remnant would no longer produce light or heat, leaving the solar system in darkness. For Earth, this would mean the end of life as we know it, as the planet would be plunged into a perpetual freeze.
Could the Core Cool Prematurely?
While the natural cooling of the core is a gradual process, scientists have speculated about scenarios that could accelerate it. For instance, if the Sun were to lose mass due to external forces, the gravitational pressure sustaining the core's temperature might weaken. However, such scenarios are purely hypothetical and unlikely to occur within the Sun's natural lifecycle.
How Does the Sun's Core Compare to Other Stars?
The Sun is often considered an "average
Mastering The Art Of Hitching A Trailer In Project Zomboid: A Comprehensive Guide
Vanessa Trump Gavin Newsom: Exploring The Lives Of Two Influential Figures
Courtney From IShowSpeed: The Rising Star You Need To Know About
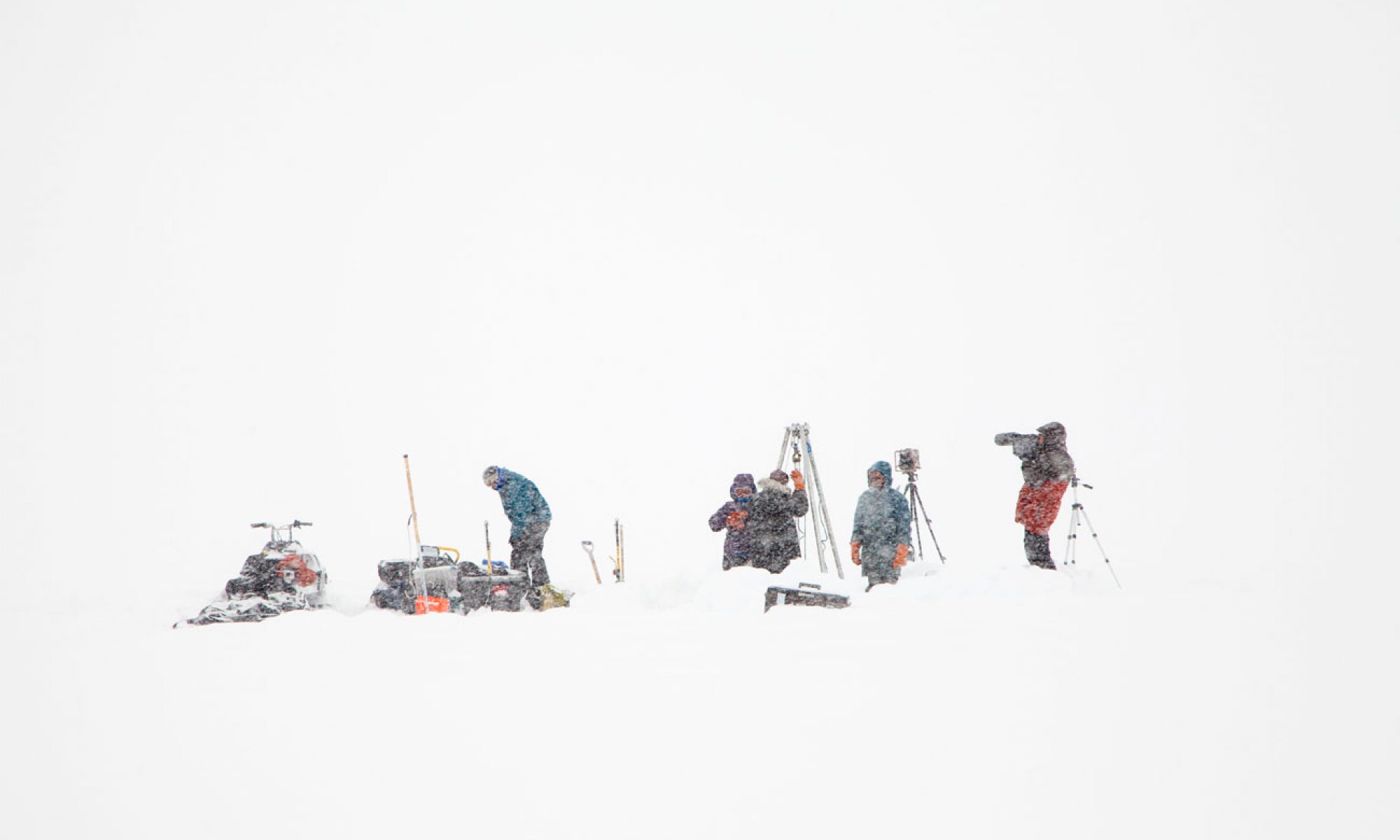
The Movie Taking Earths Temperature
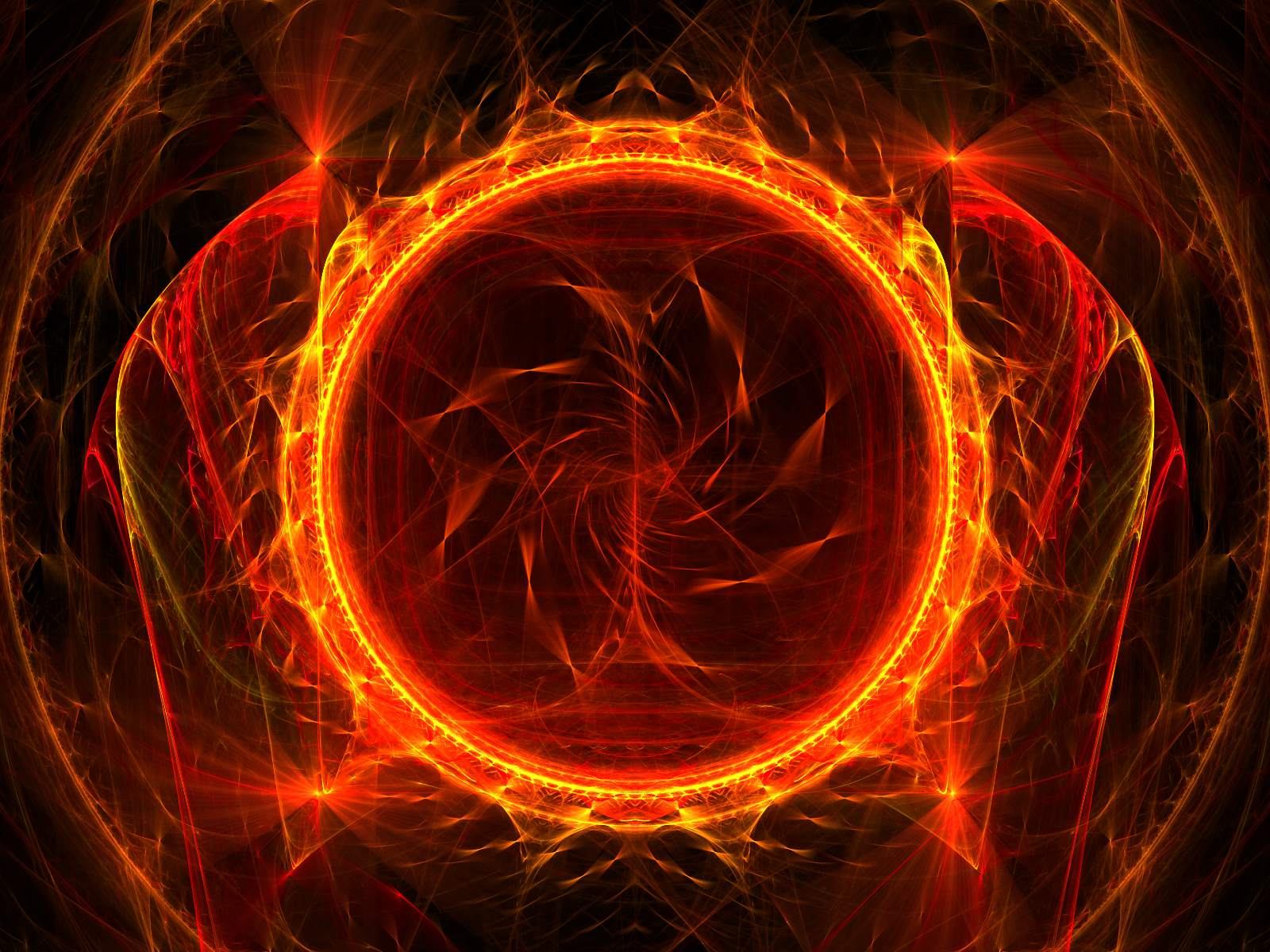
Core Of The Sun by MurdocSnook on DeviantArt